Flue gas desulfurization: Difference between revisions
imported>Milton Beychok (New page: {{subpages}} Category:CZ Live) |
imported>Milton Beychok (Created new article) |
||
Line 1: | Line 1: | ||
{{subpages}} | {{subpages}} | ||
[[Image:Four Corners Power Plant.jpg|right|thumb|250px|{{#ifexist:Template:Four Corners Power Plant.jpg/credit|{{Four Corners Power Plant.jpg/credit}}<br/>|}}Before flue gas desulfurization was installed, the emissions from this power plant in [[New Mexico]] contained excessive amounts of [[sulfur dioxide]]]] | |||
'''Flue gas desulfurization''' (FGD) is the technology used for removing [[sulfur dioxide]] (SO<sub>2</sub>) from the exhaust [[flue gases]] of [[power plant]]s that burn coal or oil to produce steam for the [[steam turbines|turbines]] that drive their electricity [[generators]]. The most common types of FGD contact the flue gases with an [[alkaline]] [[sorbent]] such as [[lime]] or [[limestone]]. <ref name=Schnelle>{{cite book|author=Karl B. Schnelle and Charles A. Brown|title=Air Pollution Control Technology>|edition= |publisher=CRC Press|year=2001|id=ISBN 0-8493-9599-7}}</ref><ref name=ICAC>[http://www.icac.com/i4a/pages/index.cfm?pageid=3401 SO<sub>2</sub> Control Technologies] (from website of the Institute of Clean Air Companies)</ref><ref name=EPA>[http://www.epa.gov/ttn/catc/dir1/ffdg.pdf Air Pollution Control Technology Fact Sheet] [[U.S. EPA]] publications EPA-452/F-03-034</ref> | |||
As sulfur dioxide is responsible for [[acid rain]] formation, stringent environmental protection regulations have been enacted in many countries to limit the amount of sulfur dioxide emissions from power plants and other industrial facilities. | |||
Prior to the advent of strict environmental protection regulations, tall flue gas stacks (i.e., chimneys) were built to disperse rather than remove the sulfur dioxide emissions. However, that only led to the transport of the emissions to other regions. For that reason, a number of countries also have regulations limiting the height of flue gas stacks. | |||
For a typical coal-fired power station, FGD technology will remove 95 percent or more of the SO<sub>2</sub> in the flue gases. | |||
==History== | |||
Methods for removing sulfur dioxide from flues gases have been studied for over 150 years. Early concepts useful for flue gas desulfurization appear to have germinated in 1850 in [[England]]. | |||
With the construction of large-scale power plants in England in the 1920s, the problems associated with large volumes of SO<sub>2</sub> emissions began to concern the public. The problem did not receive much attention until 1929, when the British government upheld the claim of a landowner against the Barton Electricity Works for damages to his land resulting from SO<sub>2</sub> emissions. Shortly thereafter a press campaign was launched against the erection of power plants within the confines of [[London]]. This led to the imposition of SO<sub>2</sub> controls on all such power plants.<ref name=Biondo>Biondo, S.J. and Marten,J.C., ''A History of Flue Gas Desulfurization Systems Since 1850'', Journal of the Air Pollution Control Association, Vol. 27, No. 10, pp 948-961, October 1977.</ref> | |||
During this period, major FGD installations went into operation in England at three power plants. The first one began operation at the Battersea Station in London in 1931. In 1935, the second one went into service at the Swansea Power Station. The third one was installed in 1938 at the Fulham Power Station. All three installations were abandoned during [[World War II]]. | |||
Large-scale FGD units did not reappear in commercial operation until the 1970s, and most of the activity occurred in the [[United States]] and [[Japan]].<ref name=Biondo/> As of June 1973, there were 42 FGD units, ranging in size from 5 to 250 [[megawatts]], in operation: 36 in Japan and 6 in the United States.<ref name=Beychok>Beychok, Milton R., ''Coping With SO<sub>2</sub>'', Chemical Engineering/Deskbook Issue, October 21, 1974</ref> | |||
As of about 1999-2000, there were 678 FGD units operating worldwide (in 27 countries) producing a total of about 229 [[gigawatts]]. About 45% of that FGD capacity was in the United States, 24% in [[Germany]], 11% in Japan and 20% in various other countries. Approximately 79% of the units, representing about 199 gigawatts of capacity, were using lime or limestone wet scrubbing. About 18% (or 25 gigawatts) utilized spray-dry scrubbers or dry sorbent injection systems.<ref name=BW>Nolan, Paul S., ''Flue Gas Desulfurization Technologies for Coal-Fired Power Plants'', The Babcock & Wilcox Company, U.S., presented by Michael X. Jiang at the Coal-Tech 2000 International Conference, November, 2000, Jakarta, Indonesia</ref><ref name=CMU>Rubin, E.S., Yeh, S., Hounsell, D.A., and Taylor, M.R., ''Experience curves for power plant emission control technologies'', Int. J. Energy Technology and Policy, Vol. 2, Nos. 1/2, 2004</ref><ref>Beychok, Milton R., ''Comparative economics of advanced regenerable flue gas desulfurization processes'', EPRI CS-1381, Electric Power Research Institute, March 1980</ref> | |||
== FGD chemistry == | |||
SO<sub>2</sub> is an acid gas. Therefore, the most common large-scale FGD systems use an alkaline sorbent such as [[lime]] or [[limestone]] to neutralize and remove the SO<sub>2</sub> from the flue gas. Since lime and limestone are not soluble in water, they are used either in the form of an aqueous slurry or in a dry, powdered form. | |||
When using an aqueous slurry of sorbent, the FGD system is referred to as a ''wet scrubber''. When using a dry, powdered sorbent, the system is referred to as a ''dry'' system. An intermediate or semi-dry system is referred to as a ''spray-dry'' system. | |||
The reaction taking place in wet scrubbing using | |||
a CaCO<sub>3</sub> (limestone) slurry produces CaSO<sub>3</sub> ([[calcium sulfite]]) and can be expressed as: | |||
:'''CaCO<sub>3</sub> (solid) + SO<sub>2</sub> (gas) → CaSO<sub>3</sub> (solid) + CO<sub>2</sub> (gas)''' | |||
When wet scrubbing with a Ca(OH)<sub>2</sub> (lime) slurry, the reaction also produces CaSO<sub>3</sub> (calcium sulfite) and can be expressed as: | |||
:'''Ca(OH)<sub>2</sub> (solid) + SO<sub>2</sub> (gas) → CaSO<sub>3</sub> (solid) + H<sub>2</sub>O (liquid)''' | |||
When wet scrubbing with a Mg(OH)<sub>2</sub> ([[magnesium hydroxide]]) slurry, the reaction produces MgSO<sub>3</sub> ([[magnesium sulfite]]) and can be expressed as: | |||
:'''Mg(OH)<sub>2</sub> (solid) + SO<sub>2</sub> (gas) → MgSO<sub>3</sub> (solid) + H<sub>2</sub>O (liquid)''' | |||
Some FGD systems go a step further and oxidize the CaSO<sub>3</sub> (calcium sulfite) to produce marketable CaSO<sub>4</sub> · 2H<sub>2</sub>O ([[gypsum]]): | |||
:'''CaSO<sub>3</sub> (solid) + ½O<sub>2</sub> (gas) + 2H<sub>2</sub>O (liquid) → CaSO<sub>4</sub> · 2H<sub>2</sub>O (solid)''' | |||
Aqueous solutions of [[sodium hydroxide]] (known as ''caustic soda'' or simply ''caustic'') may also be used to neutralize and remove SO<sub>2</sub> from flue gases. However, caustic soda is limited to small-scale FGD systems, mostly in industrial facilities other than power plants because it is more expensive than lime. It has the advantage that it forms a solution rather than a slurry and that makes it easier to operate. It produces a solution of [[sodium sulfite]] or [[sodium bisulfite]] (depending on the [[pH]]), or [[sodium sulfate]] that must be disposed of. This is not a problem in a paper mill for example, where the solution can be recycled and reused within the paper mill. | |||
== Types of FGD systems == | |||
The major types of large-scale, power plant FGD systems include spray towers, spray dryers and dry sorbent injection systems. | |||
===Spray tower=== | |||
[[Image:FGD Locations.png|right|thumb|274px|{{#ifexist:Template:FGD Locations.png/credit|{{FGD Locations.png/credit}}<br/>|}}Location of the various FGD options]] | |||
There are various types of wet scrubbers. For example, spray towers, [[venturi]] scrubbers, packed towers and trayed towers. Slurries would cause serious erosion problems in a venturi scrubber because of the high speeds at the throat of the venturi section. Packed towers or trayed towers would plug up if handling slurries. For handling slurries, the spray tower is a good choice and it is in fact a commonly used choice in large-scale FGD systems.<ref name=EPA/><ref> | |||
[http://www.babcock.com/library/pdf/br-1643.pdf Wet FGD System Materials Cost Update, by M.G. Milobowski, Babcock & Wilcox] (Presented at EPRI-DOE-EPA Combined Utility Air Pollutant Control | |||
Symposium, August 1997)</ref><ref name=EPA2>[http://www.epa.gov/ttncatc1/dir1/fsprytwr.pdf Air Pollution Control Technology Fact Sheet] [[U.S. EPA]] publications EPA-452/F-03-016</ref> | |||
Spray towers are used downstream of the particulate equipment ([[electrostatic precipitator]] or [[baghouse]]) where the flue gas contains very little, if any, combustion [[fly ash]]. In a spray tower system, the sorbent slurry is simply injected via spray nozzles into a vertical tower where the slurry droplets are contacted with the upflowing flue gas. | |||
Part of the water in the slurry is evaporated by the hot flue gas and the flue gas becomes saturated with water vapor. | |||
The SO<sub>2</sub> dissolves into the slurry droplets and reacts with the alkaline sorbent particles. The slurry falls to the bottom of the spray tower and is sent to a reaction tank where the reaction is completed and a neutral salt is formed. In a regenerable system, the residual slurry is recycled back for reuse in the spray tower. In a once-through system, the residual slurry is dewatered and either disposed of or oxidized to CaSO<sub>4</sub> · 2H<sub>2</sub>O and sold as a by-product gypsum. | |||
===Spray-dryer=== | |||
Spray-dryers are used upstream of the particulate removal equipment (electrical precipitator or baghouse) where the flue gas contains the combustion fly ash. In a spray-dryer system, the alkaline sorbent is usually lime slurry. The slurry is atomized and sprayed into a vessel as a cloud of fine bubbles where it contacts the hot flue gas. The water is completely evaporated by the hot gas and the residence time in the vessel (about 10 seconds) allows the SO<sub>2</sub> and any other acid gases, such as SO<sub>3</sub> and HCl, to react with the lime to form a dry powder of calcium sulfite, calcium sulfate and unreacted lime.<ref name=EPA/><ref name=IEA>[http://www.iea-coal.org.uk/content/default.asp?PageID=1002 IEA Clean Coal Center: Spray dry scrubbers for SO<sub>2</sub> removal]</ref><ref name=Babcock2>[http://www.babcock.com/library/pdf/E1013178.pdf Dry Flue Gas Desulfurization (FGD)Systems] (From Babcock and Wilcox website)</ref> | |||
The dry powder is removed from the flue gas along with the combustion fly ash in the [[particulate matter|particulate]] removal equipment. Some of the solids from the particulate removal equipment (i.e., fly ash, calcium sulfite, calcium sulfate and unreacted lime) may be recycled and reused as part of the sorbent slurry.<ref name=Babcock2/> | |||
===Dry sorbent injection=== | |||
The dry FGD system simply injects powdered lime or limestone sorbent directly into the flue gas. As shown in the adjacent location diagram, the dry sorbent may be injected into any one of three locations: (1) the upper section of the [[steam generator]], (2) the [[economizer]] section of the steam generator or the ducting between the [[air preheater]] and the electrostatic precipitator.<ref name=ICAC/><ref name=EPA/><ref name=IEA/><ref>{{cite book|author=Barbara Toole-O'Neil and Ohio Coal Development Office (Editors)|title=Dry Scrubbing Technologies for Flue Gas Desulfurization|edition=|publisher=Springer|year=1998|id=ISBN 0-7923-8346-X}}</ref> | |||
The powdered sorbent is pneumatically injected through lances designed to distribute the sorbent evenly across the flow path of the flue gas. | |||
When injected into the upper section of the steam generator, it should enter at a point where the temperature range is about 900 to 1200 °C. Injection into the economizer should be at a point where the temperature range is about 400 to 600 °C. Injection into the ducting between the preheater and the precipitator should be at point where the flue gas temperature is about 150 to 180 °C.<ref name=EPA/><ref name=IEA/> | |||
The SO<sub>2</sub> reacts directly with the powdered sorbent and the spent sorbent is removed from the flue gas along with the combustion fly ash in the [[particulate matter|particulate]] removal equipment | |||
==Facts and statistics== | |||
:''The information in this section was obtained from a [[U.S. EPA]] published fact sheet.''<ref name=EPA/> | |||
Flue gas desulfurization scrubbers have been applied to combustion units firing coal and oil that range in size from 5 MW to 1500 MW. Scottish Power are spending £400 million installing FGD at Longannet power station which has a capacity of over 2 GW. Dry scrubbers and spray scrubbers have generally been applied to units smaller than 300 MW. | |||
Approximately 85% of the flue gas desulfurization units installed in the US are wet scrubbers, 12% are spray dry systems and 3% are dry injection systems. | |||
The highest SO2 removal efficiencies (greater than 90%) are achieved by wet scrubbers and the lowest (less than 80%) by dry scrubbers. However, the newer designs for dry scrubbers are capable of achieving efficiencies in the order of 90%. | |||
The capital, operating and maintenance costs per [[short ton]] of SO<sub>2</sub> removed (in 2001 US dollars) are: | |||
*For wet scrubbers larger than 400 MW, the cost is $200 to $500 per ton | |||
*For wet scrubbers smaller than 400 MW, the cost is $500 to $5,000 per ton | |||
*For spray dry scrubbers larger than 200 MW, the cost is $150 to $300 per ton | |||
*For spray dry scrubbers smaller than 200 MW, the cost is $500 to $4,000 per ton | |||
== Alternative methods of reducing sulfur dioxide emissions == | |||
An alternative to removing [[sulfur]] from the flue gases after burning is to remove the sulfur from the fuel before or during combustion. [[Hydrodesulfurization]] of fuel has been used for treating [[fuel oil]]s. | |||
[[Fluidized bed combustion]] adds lime to the fuel during combustion. The lime reacts with the SO<sub>2</sub> to form [[sulfate]]s which become part of the combustion ash. | |||
== Sulfuric acid mist formation == | |||
[[Fossil fuel]]s such as coal and oil contain significant amounts of sulfur. When burned, about 95 percent or more of the sulfur is generally converted to sulfur dioxide (SO<sub>2</sub>). This happens under normal conditions of temperature and of oxygen present in the flue gas. However, there are circumstances under which this may not be the case. | |||
For example, when the flue gas has too much [[oxygen]] and the SO<sub>2</sub> is further oxidized to [[sulfur trioxide]] (SO<sub>3</sub>). Actually, too much oxygen is only one of the ways that SO<sub>3</sub> is formed. Gas temperature is also an important factor. At about 800 °C, formation of SO<sub>3</sub> is favored. Another way that SO<sub>3</sub> can be formed is through catalysis by trace metals in the fuel. This is particularly true for heavy fuel oil, where small amounts of [[vanadium]] are present. In whatever way that SO<sub>3</sub> is formed, it does not behave like SO<sub>2</sub> in that it forms a liquid [[aerosol]] known as [[sulfuric acid]] (H<sub>2</sub>SO<sub>4</sub>) mist that is very difficult to remove. Generally, about 1% of the sulfur dioxide will be converted to SO<sub>3</sub>. Since SO<sub>3</sub> an acid gas, just as is SO<sub>2</sub>, it is also removed by the alkaline sorbents used in FGD systems. | |||
==References== | |||
{{reflist}} | |||
[[Category:CZ Live]] | [[Category:CZ Live]] |
Revision as of 00:33, 20 February 2008

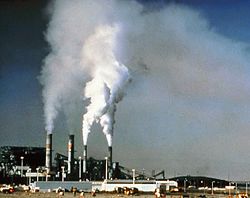
Before flue gas desulfurization was installed, the emissions from this power plant in New Mexico contained excessive amounts of sulfur dioxide
Flue gas desulfurization (FGD) is the technology used for removing sulfur dioxide (SO2) from the exhaust flue gases of power plants that burn coal or oil to produce steam for the turbines that drive their electricity generators. The most common types of FGD contact the flue gases with an alkaline sorbent such as lime or limestone. [1][2][3] As sulfur dioxide is responsible for acid rain formation, stringent environmental protection regulations have been enacted in many countries to limit the amount of sulfur dioxide emissions from power plants and other industrial facilities.
Prior to the advent of strict environmental protection regulations, tall flue gas stacks (i.e., chimneys) were built to disperse rather than remove the sulfur dioxide emissions. However, that only led to the transport of the emissions to other regions. For that reason, a number of countries also have regulations limiting the height of flue gas stacks.
For a typical coal-fired power station, FGD technology will remove 95 percent or more of the SO2 in the flue gases.
History
Methods for removing sulfur dioxide from flues gases have been studied for over 150 years. Early concepts useful for flue gas desulfurization appear to have germinated in 1850 in England.
With the construction of large-scale power plants in England in the 1920s, the problems associated with large volumes of SO2 emissions began to concern the public. The problem did not receive much attention until 1929, when the British government upheld the claim of a landowner against the Barton Electricity Works for damages to his land resulting from SO2 emissions. Shortly thereafter a press campaign was launched against the erection of power plants within the confines of London. This led to the imposition of SO2 controls on all such power plants.[4]
During this period, major FGD installations went into operation in England at three power plants. The first one began operation at the Battersea Station in London in 1931. In 1935, the second one went into service at the Swansea Power Station. The third one was installed in 1938 at the Fulham Power Station. All three installations were abandoned during World War II.
Large-scale FGD units did not reappear in commercial operation until the 1970s, and most of the activity occurred in the United States and Japan.[4] As of June 1973, there were 42 FGD units, ranging in size from 5 to 250 megawatts, in operation: 36 in Japan and 6 in the United States.[5]
As of about 1999-2000, there were 678 FGD units operating worldwide (in 27 countries) producing a total of about 229 gigawatts. About 45% of that FGD capacity was in the United States, 24% in Germany, 11% in Japan and 20% in various other countries. Approximately 79% of the units, representing about 199 gigawatts of capacity, were using lime or limestone wet scrubbing. About 18% (or 25 gigawatts) utilized spray-dry scrubbers or dry sorbent injection systems.[6][7][8]
FGD chemistry
SO2 is an acid gas. Therefore, the most common large-scale FGD systems use an alkaline sorbent such as lime or limestone to neutralize and remove the SO2 from the flue gas. Since lime and limestone are not soluble in water, they are used either in the form of an aqueous slurry or in a dry, powdered form.
When using an aqueous slurry of sorbent, the FGD system is referred to as a wet scrubber. When using a dry, powdered sorbent, the system is referred to as a dry system. An intermediate or semi-dry system is referred to as a spray-dry system.
The reaction taking place in wet scrubbing using a CaCO3 (limestone) slurry produces CaSO3 (calcium sulfite) and can be expressed as:
- CaCO3 (solid) + SO2 (gas) → CaSO3 (solid) + CO2 (gas)
When wet scrubbing with a Ca(OH)2 (lime) slurry, the reaction also produces CaSO3 (calcium sulfite) and can be expressed as:
- Ca(OH)2 (solid) + SO2 (gas) → CaSO3 (solid) + H2O (liquid)
When wet scrubbing with a Mg(OH)2 (magnesium hydroxide) slurry, the reaction produces MgSO3 (magnesium sulfite) and can be expressed as:
- Mg(OH)2 (solid) + SO2 (gas) → MgSO3 (solid) + H2O (liquid)
Some FGD systems go a step further and oxidize the CaSO3 (calcium sulfite) to produce marketable CaSO4 · 2H2O (gypsum):
- CaSO3 (solid) + ½O2 (gas) + 2H2O (liquid) → CaSO4 · 2H2O (solid)
Aqueous solutions of sodium hydroxide (known as caustic soda or simply caustic) may also be used to neutralize and remove SO2 from flue gases. However, caustic soda is limited to small-scale FGD systems, mostly in industrial facilities other than power plants because it is more expensive than lime. It has the advantage that it forms a solution rather than a slurry and that makes it easier to operate. It produces a solution of sodium sulfite or sodium bisulfite (depending on the pH), or sodium sulfate that must be disposed of. This is not a problem in a paper mill for example, where the solution can be recycled and reused within the paper mill.
Types of FGD systems
The major types of large-scale, power plant FGD systems include spray towers, spray dryers and dry sorbent injection systems.
Spray tower
There are various types of wet scrubbers. For example, spray towers, venturi scrubbers, packed towers and trayed towers. Slurries would cause serious erosion problems in a venturi scrubber because of the high speeds at the throat of the venturi section. Packed towers or trayed towers would plug up if handling slurries. For handling slurries, the spray tower is a good choice and it is in fact a commonly used choice in large-scale FGD systems.[3][9][10]
Spray towers are used downstream of the particulate equipment (electrostatic precipitator or baghouse) where the flue gas contains very little, if any, combustion fly ash. In a spray tower system, the sorbent slurry is simply injected via spray nozzles into a vertical tower where the slurry droplets are contacted with the upflowing flue gas.
Part of the water in the slurry is evaporated by the hot flue gas and the flue gas becomes saturated with water vapor.
The SO2 dissolves into the slurry droplets and reacts with the alkaline sorbent particles. The slurry falls to the bottom of the spray tower and is sent to a reaction tank where the reaction is completed and a neutral salt is formed. In a regenerable system, the residual slurry is recycled back for reuse in the spray tower. In a once-through system, the residual slurry is dewatered and either disposed of or oxidized to CaSO4 · 2H2O and sold as a by-product gypsum.
Spray-dryer
Spray-dryers are used upstream of the particulate removal equipment (electrical precipitator or baghouse) where the flue gas contains the combustion fly ash. In a spray-dryer system, the alkaline sorbent is usually lime slurry. The slurry is atomized and sprayed into a vessel as a cloud of fine bubbles where it contacts the hot flue gas. The water is completely evaporated by the hot gas and the residence time in the vessel (about 10 seconds) allows the SO2 and any other acid gases, such as SO3 and HCl, to react with the lime to form a dry powder of calcium sulfite, calcium sulfate and unreacted lime.[3][11][12]
The dry powder is removed from the flue gas along with the combustion fly ash in the particulate removal equipment. Some of the solids from the particulate removal equipment (i.e., fly ash, calcium sulfite, calcium sulfate and unreacted lime) may be recycled and reused as part of the sorbent slurry.[12]
Dry sorbent injection
The dry FGD system simply injects powdered lime or limestone sorbent directly into the flue gas. As shown in the adjacent location diagram, the dry sorbent may be injected into any one of three locations: (1) the upper section of the steam generator, (2) the economizer section of the steam generator or the ducting between the air preheater and the electrostatic precipitator.[2][3][11][13]
The powdered sorbent is pneumatically injected through lances designed to distribute the sorbent evenly across the flow path of the flue gas.
When injected into the upper section of the steam generator, it should enter at a point where the temperature range is about 900 to 1200 °C. Injection into the economizer should be at a point where the temperature range is about 400 to 600 °C. Injection into the ducting between the preheater and the precipitator should be at point where the flue gas temperature is about 150 to 180 °C.[3][11]
The SO2 reacts directly with the powdered sorbent and the spent sorbent is removed from the flue gas along with the combustion fly ash in the particulate removal equipment
Facts and statistics
Flue gas desulfurization scrubbers have been applied to combustion units firing coal and oil that range in size from 5 MW to 1500 MW. Scottish Power are spending £400 million installing FGD at Longannet power station which has a capacity of over 2 GW. Dry scrubbers and spray scrubbers have generally been applied to units smaller than 300 MW.
Approximately 85% of the flue gas desulfurization units installed in the US are wet scrubbers, 12% are spray dry systems and 3% are dry injection systems.
The highest SO2 removal efficiencies (greater than 90%) are achieved by wet scrubbers and the lowest (less than 80%) by dry scrubbers. However, the newer designs for dry scrubbers are capable of achieving efficiencies in the order of 90%.
The capital, operating and maintenance costs per short ton of SO2 removed (in 2001 US dollars) are:
- For wet scrubbers larger than 400 MW, the cost is $200 to $500 per ton
- For wet scrubbers smaller than 400 MW, the cost is $500 to $5,000 per ton
- For spray dry scrubbers larger than 200 MW, the cost is $150 to $300 per ton
- For spray dry scrubbers smaller than 200 MW, the cost is $500 to $4,000 per ton
Alternative methods of reducing sulfur dioxide emissions
An alternative to removing sulfur from the flue gases after burning is to remove the sulfur from the fuel before or during combustion. Hydrodesulfurization of fuel has been used for treating fuel oils.
Fluidized bed combustion adds lime to the fuel during combustion. The lime reacts with the SO2 to form sulfates which become part of the combustion ash.
Sulfuric acid mist formation
Fossil fuels such as coal and oil contain significant amounts of sulfur. When burned, about 95 percent or more of the sulfur is generally converted to sulfur dioxide (SO2). This happens under normal conditions of temperature and of oxygen present in the flue gas. However, there are circumstances under which this may not be the case.
For example, when the flue gas has too much oxygen and the SO2 is further oxidized to sulfur trioxide (SO3). Actually, too much oxygen is only one of the ways that SO3 is formed. Gas temperature is also an important factor. At about 800 °C, formation of SO3 is favored. Another way that SO3 can be formed is through catalysis by trace metals in the fuel. This is particularly true for heavy fuel oil, where small amounts of vanadium are present. In whatever way that SO3 is formed, it does not behave like SO2 in that it forms a liquid aerosol known as sulfuric acid (H2SO4) mist that is very difficult to remove. Generally, about 1% of the sulfur dioxide will be converted to SO3. Since SO3 an acid gas, just as is SO2, it is also removed by the alkaline sorbents used in FGD systems.
References
- ↑ Karl B. Schnelle and Charles A. Brown (2001). Air Pollution Control Technology>. CRC Press. ISBN 0-8493-9599-7.
- ↑ Jump up to: 2.0 2.1 SO2 Control Technologies (from website of the Institute of Clean Air Companies)
- ↑ Jump up to: 3.0 3.1 3.2 3.3 3.4 3.5 Air Pollution Control Technology Fact Sheet U.S. EPA publications EPA-452/F-03-034
- ↑ Jump up to: 4.0 4.1 Biondo, S.J. and Marten,J.C., A History of Flue Gas Desulfurization Systems Since 1850, Journal of the Air Pollution Control Association, Vol. 27, No. 10, pp 948-961, October 1977.
- ↑ Beychok, Milton R., Coping With SO2, Chemical Engineering/Deskbook Issue, October 21, 1974
- ↑ Nolan, Paul S., Flue Gas Desulfurization Technologies for Coal-Fired Power Plants, The Babcock & Wilcox Company, U.S., presented by Michael X. Jiang at the Coal-Tech 2000 International Conference, November, 2000, Jakarta, Indonesia
- ↑ Rubin, E.S., Yeh, S., Hounsell, D.A., and Taylor, M.R., Experience curves for power plant emission control technologies, Int. J. Energy Technology and Policy, Vol. 2, Nos. 1/2, 2004
- ↑ Beychok, Milton R., Comparative economics of advanced regenerable flue gas desulfurization processes, EPRI CS-1381, Electric Power Research Institute, March 1980
- ↑ Wet FGD System Materials Cost Update, by M.G. Milobowski, Babcock & Wilcox (Presented at EPRI-DOE-EPA Combined Utility Air Pollutant Control Symposium, August 1997)
- ↑ Air Pollution Control Technology Fact Sheet U.S. EPA publications EPA-452/F-03-016
- ↑ Jump up to: 11.0 11.1 11.2 IEA Clean Coal Center: Spray dry scrubbers for SO2 removal
- ↑ Jump up to: 12.0 12.1 Dry Flue Gas Desulfurization (FGD)Systems (From Babcock and Wilcox website)
- ↑ Barbara Toole-O'Neil and Ohio Coal Development Office (Editors) (1998). Dry Scrubbing Technologies for Flue Gas Desulfurization. Springer. ISBN 0-7923-8346-X.